Stainless steel is an essential material that underpins countless industries, from construction and automotive to medical and aerospace applications. However, not all stainless steel is created equal — its performance, durability, and suitability for specific environments vary greatly depending on its composition and structure. Two of the most commonly used types are austenitic and ferritic stainless steel, each offering distinctive characteristics that cater to different needs. This article is designed to demystify the key differences between these two stainless steel categories, providing you with a comprehensive understanding of their unique properties, benefits, and real-world applications. Whether you are a materials engineer, a manufacturer, or simply curious about industrial materials, this guide will equip you with the knowledge to make informed decisions when it comes to stainless steel selection.
What is Austenitic Stainless Steel?
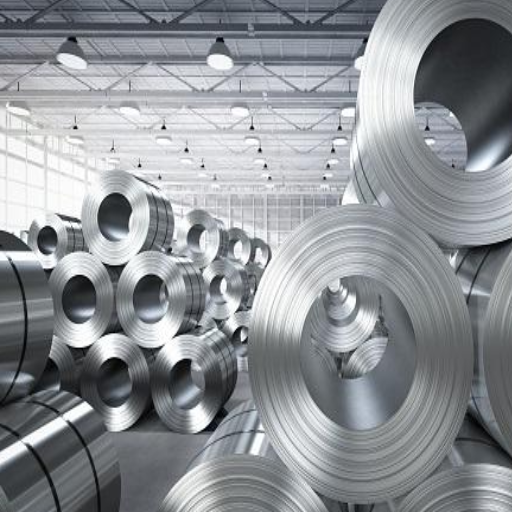
Characteristics of Austenitic Stainless Steel
The most utilized type of stainless steel is austenitic stainless steel because of its high corrosion resistance, formability, and weldability. The high corrosion resistance of austenitic stainless steel is because of the iron, chromium, and nickel present in it, which typically has more than 16% chromium and 6% nickel. These materials prevent the moisture, air, and chemicals from coming in contact with it, thanks to the passive oxide layer formed on its surface. For chemical plants, marine units, and food processing industries, austenitic stainless steel works the best.
In the annealed state, austenitic stainless steel is known to be non-magnetic and stays that way throughout the process. Its outstanding strength and toughness even at cryogenic temperatures make it suitable for the construction of cryogenic tanks, heat exchangers, and medical machinery. Exposing austenitic stainless steel to extreme heat and cold does not alter its mechanical properties, making it reliable.
It is also famous for its ease of fabrication. Compared to other grades of stainless steel, austenitic ones are simpler to shape and weld without loss of strength or durability. Common cases include stainless steel 304 and 316 grades, as they are famous for their versatile uses. On the contrary, one downside of austenitic stainless steel is how expensive it is relative to other steel types because of its higher nickel content; thus, its benefits have to be weighed against the demands of the intended application.
Common Applications and Uses
Austenitic stainless steels 304 and 316 are critically industrial and commercially important due to their remarkable corrosion resistance, weldability, and strength. These grades have remarkable resistance toward food acids and can be sanitized effectively thus are used extensively in the food and beverage industry. These grades are used for making kitchen equipment like sinks, cookware as well as food processing machinery. Because they are non-reactive, austenitic stainless steels also help in preserving the safety and purity of the consumable product.
Another notable area of use is in healthcare. Austenitic stainless steels are common in the fabrication of surgical tools, medical devices, and implants. Grade 316, for example, is frequently selected for its enhanced resistance to saline environments, making it applicable for surgical and marine use. Their ability to endure repeated sterilization processes also makes them much more useful in healthcare.
Moreover, austenitic stainless steels are essential for construction and architecture. They are commonly utilized in structural parts, handrails, and exterior cladding because of their aesthetic value and lasting appeal. Also, their resistance to different environmental factors like moist conditions and temperature changes makes them suitable for long-term infrastructure undertakings. This adaptability shows why these grades continue to be the material of choice in challenging applications throughout different industries.
Benefits of Using Austenitic Stainless Steel
One of the most important benefits of austenitic stainless steel is its exceptional corrosion resistance. Austenitic stainless steel is ideal for parts that are exposed to moisture, chemicals, and high temperatures due to the chromium-nickel structure that enables passive oxidation. Austenitic stainless steel is often used in construction, marine, and chemical industry applications that are termed as critical; this is due to their need for enduring robust mechanical durability and strength.
Austenitic stainless steel also possesses excellent mechanical properties, ranging from strength to ductility. Strength and ductility allow smelling to withstand significant levels stress and deformation and still retain functionality, making the material ideal for stable structural and load-bearing applications. Not just that, Austenitic stainless steel remains remarkably tough even under high and low temperatures, this, along with its use for cryogenic storage tanks and heat exchangers, makes it extremely versatile.
Austenitic stainless steel is equally flexible when it comes to fabrication and finishing techniques. It can be readily welded, easily machined, and formed into intricate shapes, thus giving manufacturers freedom to cater to some tailored solutions for particular design needs. Additionally, the material has a highly polishable surface and a pleasing look, which contributes to its frequent use in construction and design projects. Along with these advantages, his type of steel has a long working life and low maintenance needs, making austenitic stainless steel cost-effective and a trustworthy answer for many industrial and commercial operations.
Exploring Ferritic Stainless Steel
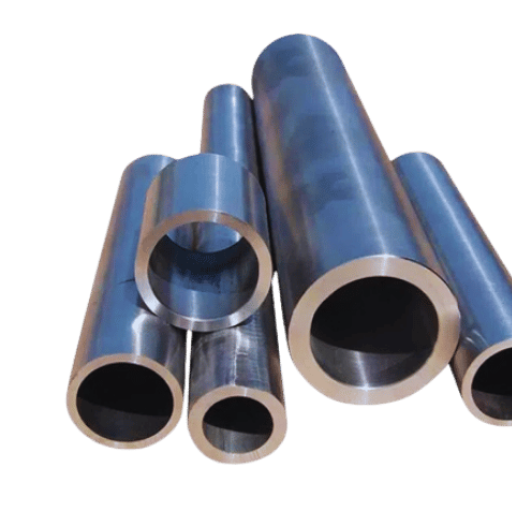
Properties of Ferritic Stainless Steel
Ferritic stainless steel is distinguished by the amount of chromium contained in it, which usually ranges from 10.5% to 30%, and nickel present in it is either very low or almost absent. This structure results in the material being well protected against oxidation and corrosion, especially in less corrosive conditions. The distinguishing features of ferritic stainless steels are their crystal structure which is body-centered cubic (BCC), this structure is associated with better thermal conductivity when compared to the austenitic grades.
Due to body-centered cubic structure, ferritic stainless steels are also associated with great resistance to SCC (stress corrosion cracking), making the alloys useful in applications where there is exposure to Chloride. With lower nickel amounts, ferritic alloys are cheaper than austenitic stainless steels especially during the periods of high nickel price volatility.
Steel possessing ferritic characteristics is not very strong. These alloys provide lower ductility, which still gets the job done with processes like forming and welding which do not harden the materials due to heating. Compared to austenitic steel, ferritic alloys lack ductility. At the same time, ferritic grades are better at resisting scaling compared to austenitic grades at high temperatures. This is particularly useful when using stainless steel for automotive exhaust systems.
Like every other type of steel used in construction, ferritic stainless steel comes with its limitations. For instance, its toughness is lower compared to alternative types of stainless steel, particularly at lower temperatures. These limitations should always be taken into advantageous consideration before handling ferritic grades for the desired tasks.
How Ferritic Alloys Differ from Other Alloys
The notable features that differentiate ferritic alloys from other types of alloys are their high chromium content, low carbon levels, lack of nickel, magnetic properties, and body-centered cubic grain formation.
I’ve captured the details of each alloy type in a simple table below:
Aspect | Ferritic | Austenitic | Martensitic |
---|---|---|---|
Cr Content (%) |
10.5-30 |
16-26 |
12-18 |
Ni Content (%) |
0-1 |
6-22 |
0-1 |
C Content (%) |
<0.2 |
<0.08 |
0.1-1.2 |
Crystal Type |
BCC |
FCC |
BCC |
Magnetism |
Yes |
No |
Yes |
Strengthening |
Cold-work |
Cold-work |
Heat-treat |
Rust Resistance |
Moderate |
High |
Low |
Thermal Exp. |
Low |
High |
Moderate |
Heat Conduct. |
High |
Moderate |
Low |
Uses |
Appliances, |
Food, Medical, |
Tools, Blades, |
|
Automotive |
Marine |
Cutlery |
Advantages and Limitations
Advantages
- Cr Content Variances: The second category contains higher amounts of chromium. Because of this, the material is better suited for marine or medical use where there is moisture or reactive substances, because it is more corrosion resistant.
- Crystal Structures: The second category FCC crystal structures also improve ductility and toughness, which helps mold the material more easily as well as resist fracturing when stressed.
- Magnetism: Materials with BCC structures and ferromagnetic properties are functional for mechanical applications, such as electromagnetic parts or sensors that need to be magnetized.
- Strengthening Mechanisms: Blades and tools that undergo high amounts of wear and tear require a harsh level of strength and hardness. Such materials are ideal as they are easily further hardened through heat treatment.
- Thermal Properties: The first category metals are low expansion and highly conductive towards heat, which is beneficial in dissipating heat in high temperature appliances or automotive systems.
Limitations
Despite the pros and the many uses for each of the material types, they all have corresponding cons that pose boundaries to their application:
- Corrosion Resistance: The first and third categories include materials with low chromium and nickel content, leading to moderate to low rust resistance, which decreases their service life in harsh environmental conditions.
- Magnetic Limitations: Metals in the second category will be non-magnetic, making them unsuitable for use in applications in which magnetic interaction is of fundamental importance, therefore limiting functionality for other cases.
- Thermal Conductivity: Dimensional stability in high temperatures is needed in the third category where materials with lower thermal conductivity or higher thermal expansion are situated.
- Process-Specific Strength: Two of the categories in question rely on cold-working to enhance properties, restricting flexibility to alter mechanical properties after the initial manufacturing, which is more energy-intensive.
- Cost vs. Performance: For industrial applications, the balance between cost and corrosion resistance becomes important, as higher amounts of nickel and chromium increase costs, but are beneficial for resistance.
Considering both the advantages and limitations ensures the optimal selection of materials tailored for specific operational requirements, furthermore ensuring peak performance while maintaining efficiency and durability.
Comparing Austenitic and Ferritic Stainless Steel
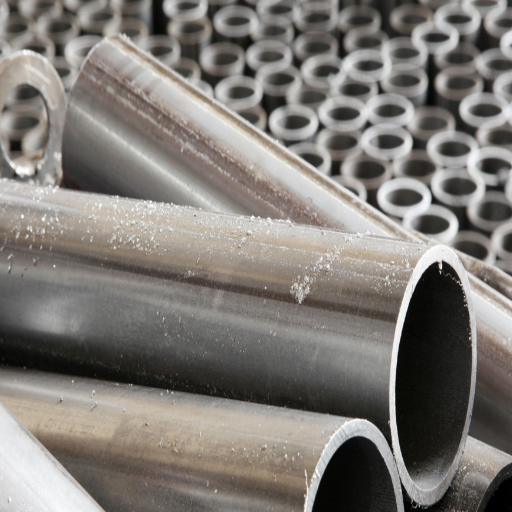
Key Differences Between Austenitic and Ferritic
The properties, application, and performance characteristics of Austenitic and ferritic stainless steels differ mainly because of their crystalline structures and they differ fundamentally in their crystalline structures. Superiority in ductility, toughness, and corrosion resistance are hallmark properties of wholly stainless austenitic steels, which stems from their Face Centered Cubic (FCC) crystal structure, high nickel and chromium content. Austenitic stainless steels are indeed a marvel of engineering, which boast impressive resistance to pitting in harsh marine environments, making them suitable for chemical processing, food-grade applications.
However, unlike Ferritic Stainless Steels, which have a lower nickel content, Ferritic Grades are more cost effective while still maintaining good corrosion resistance even in less aggressive environments. Their body-centered cubic structure grants them higher strength as compared to Austenitic, but also means lower ductility. These materials are primarily used in manufacturing automotive components, industrial equipment, and decorative items, devoid of welding concerns.
In terms of thermal performance grade, Ferritic Stainless Steels possess superior thermal expansion and higher thermal conductivity, making them more suitable for applications which require heat resistance, like automotive brisk exhaust systems. However, unlike their austenitic grade peers, FCCC stainless steel maintains its mechanical and corrosion-resistant properties across a wider temperature range, which makes it advantageous in extreme cryogenic and high-temperature environments.
The differences in weldability are also crucial during the selection of materials for certain applications. For example, austenitic steels do not need preheat or post-heat processes and have high weldability, whereas ferritic steels are weldable but tend to suffer from increased grain growth and lower impact toughness within the heat-affected zone, requiring more care during fabrication processes.
Knowing these differences is important for meeting all the parameters of material selection and is highly beneficial for cost and performance optimization across numerous applications.
Understanding Corrosion Resistance
Corrosion resistance is a key attribute of materials that withstand conditions of constant exposure to moisture, chemicals, or extreme temperature for any duration of time. The failure of a material to withstand the degrading reactions through electrochemical processes associated with the surroundings will determine the life span, performance, and serviceability of various industrial parts. Some of the principal contributors that affect the strength towards corrosion include the chemical nature of the material, its microstructure, and the presence of protective coatings or some other external layers on the surface.
Stainless steels are an example that depends on the formation of a passive chromium oxide layer in the presence of oxygen, which offers self-healing properties, hence increasing their corrosion resistance. Other alloys, such as super and duplex austenitic stainless steel, are observed to have improved resistance to pitting and crevice corrosion in sea water and highly acidic fluids due to higher content of chromium and molybdenum, which aids in anti-corrosive properties. Also, nonferrous alloys like Aluminum and Titanium rely on their oxide layers in resisting corrosion, while nickel alloys face most of the issues associated with uniform and localized corrosion.
This variability can be better understood using values. For instance, the loss of steel’s integrity due to corrosion can be determined using the pitting resistance equivalent number (PREN). Steel that is classified as super duplex stainless steel, for instance, has a PREN value higher than 40, which demonstrates stronger resilience. Such metrics are critical when choosing materials for sophisticated tasks like offshore drilling, chemical processing, and desalination projects, where resilience to corrosion is imperative to prevent fatal consequences. To devise methods to mitigate corrosion for materials used in specific industries, the relationships between material features, design factors, and environmental elements must be deeply understood.
Impact of Chromium Content and Nickel
The corrosion resistance as well as the mechanical properties of stainless steel, or any other advanced materials, are significantly influenced by the presence of alloys like chromium and nickel. Chromium gives a passive oxide covering on the surface of the material that ranges between 10% and 30% in amount. This self-healing covering protects the material against oxidation and corrosion. Hence, it becomes possible for the material to endure rugged environments that include high chemical agents, humidity, and saline conditions.
Nickel contributes to the austenitic structure of stainless steels by adding toughness as well as ductility to the material. Austenitic grade stainless steel 304 or 316 contains high amounts of Nickel and serves as an excellent defender against stress corrosion cracking in chloride-abundant conditions. Pitting and crevice corrosion are other forms of corrosion that are countered greatly by the nickel-containing molybdenum in 316 stainless steel, which contains 10%-14% Nickel.
New developments propose that the synergistic interaction between chromium and nickel can be optimized for designated industrial uses. Research suggests that increasing the amount of chromium improves resistance to oxidizing agents, while the presence of nickel enhances performance in reducing environments. These relationships is critically important for industries like chemical processing and marine engineering in choosing the most suitable materials which can withstand prolonged service life in extreme conditions.
The Role of Microstructure in Steel Grades
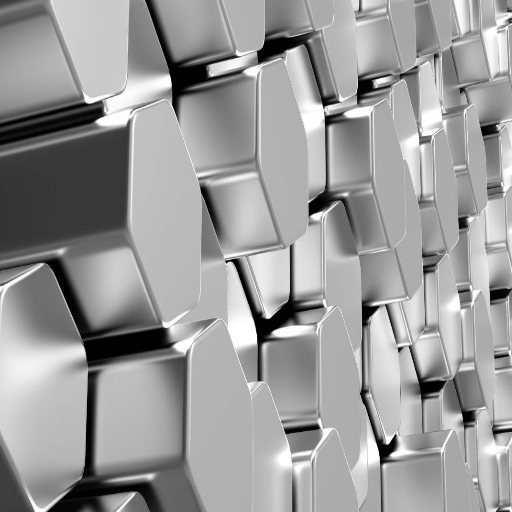
Understanding Crystalline Structure
When I tackle the topic of crystalline structure, I frame it in my head as the ‘building block’ of any material. This describes the atomic arrangement of a substance, which is important on a mechanical and chemical level. For instance, the crystalline structure in various grades of steel influences the strength and ductility, corrosion resistance, as well as the response to heat treatment. A steel product can be engineered to meet specific industrial requirements through the atomic configuration control processes.
The three most relevant to steel are body-centered cubic (BCC), face-centered cubic (FCC), and hexagonal close-packed (HCP). Each configuration offers distinct characteristics; BCC structures are typically harder and less ductile due to their lower packing efficiency while FCC structures provide superior ductility and toughness owing to their increased atomic slip systems. All these facts demonstrate the need to select proper crystalline structures for use in construction, aerospace, energy and other performance grade sensitive engineering disciplines.
Crystalline structures demand exact heat treatments and alloying practices to cater to the rich requirements of the intended use. For instance, transforming FCC austenite phase to BCC martensite phase through quenching increases hardness, although it may reduce ductility. In my opinion, this understanding of crystalline behavior enables the fundamental building knowledge of material science which facilitates advancements in the design and use of modern steel grades in various challenging industrial applications.
How Microstructure Affects Mechanical Properties
The microstructure of any given material determines its mechanical properties, such as its strength, ductility, toughness, and hardness. Structural characteristics like grain size, phase distribution, dislocation density, and precipitate morphology affect the material behavior under mechanical loading. For instance, fine micrograins produced by thermomechanical treatment or controlled solidification processes increase yield strength through the Hall-Petch relationship becausethe increase in grain boundaries decrease dislocation motion. On the other hand, coarse grains can improve ductility but may severely lower strength.
Other examples include phase changes like the transformation from austenite (FCC) to martensite (BCT), which is important in steels. Martensitic structures are very hardenable because of their distorted lattice; however, if improperly tempered, they can become brittle. Also, adding second phase particles like carbides and nitrides into a matrix improves resistance to wearing and increases load-bearing capacity, but these particles can become cracks, which make them very fragile and reduce fracture toughness.
The development of computational materials science and the use of high-resolution microscopy have facilitated quantifiable evaluation of microstructure-property relations, which further increases innovation in design. Moreover, techniques such as additive manufacturing and severe plastic deformation are providing unparalleled opportunities for microstructural refinement, permitting specific properties for particular uses in the aerospace, automotive, and biomedical industries. Hence, to manipulate microstructure is to engineer materials to withstand formidable performance requirements.
Additional Considerations: Martensitic Stainless Steel
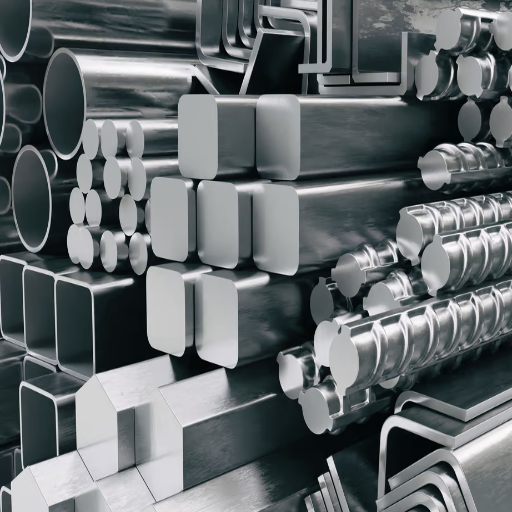
Differences with Martensitic Stainless Steel
Martensitic stainless steels estruturas martensiticas (ferritic) or (austenitic) have a distinguishing mark when compared to the other types. This is because its crystal formation is different, thus giving it those features. As a result of the three reasons together (stainless quenching formation, the strength and hardness achieved through martensite, both of which stem from the martensitic crystal structure quenching process). In comparison with other stainless steels, martensitic grades typically lack nickel but instead have lower chromium content, thus resulting in moderate corrosion resistance.
Martensitic grades’ advantage does not rest here, they are also highly responsive to wear-resistant and impact maintenance resilient applications that require mechanical hardening or thermal timidите. This creates room for structural manipulation in any manner needed through terms such as heating, tempering, or even hardening. But even with those advantages, there are a lot of shortcomings, including brittleness due to wrong treatment and drastic impact on toughness at low temperatures. Stress corrosion cracking risk also rises due to martensitic steel microstructure, therefore, the steel placement design process needs to be done around design and processing for critical applications.
Martensitic stainless steel is often used for high precision medical instruments, turbine blades, and cutting tools due to their high strength. Alloy variants like 410, 420, and 440C have different combinations of hardness, ductility, corrosion resistance, and thus enable engineers to tailor the composition to the operating conditions.
Applications of Martensitic vs Austenitic and Ferritic
The unique characteristics of martensitic, austenitic, and ferritic stainless steels allow the materials to be utilized for different purposes. With regard to their distinct microscopic structures and compositions, engineers have the option to choose the type that satisfies the environmental and mechanical requirements of a particular application.
- Medical Instruments (Martensitic): Parts like scalpels and dental equipment require precision grade stainless steels which provide excellent martensitic corrosion resistance, making 410 and 420 grade widely used. In orthopedics, these grades are used in surgical implants as well. The heat-treatable grade provides dependable and accurate results because of its hardness and ferrous material oxidation resistance.
- Chemical Processing Equipment (Austenitic): Fights off rust easily, a characteristic of 304 and 316 grades, makes them staples of chemical processing equipment and with the ability to resist acids, chlorides, and other aggressive chemicals, makes the use of austenitic grades preferable in these changing environments. These are found in pipes, storage tanks, reactors, and even in military vessels.
- Exhaust Systems (Ferritic): With the capability of enduring great amounts of stress, farrisitic stainless steels 409 grade make for long lasting automobile exhaus systems and mufflers. These are reliable during long term use and fortify resistance to stress ferrous material corrosion cracking as well as oxidation at elevated temperatures.
- Turbine Blades (Martensitic): In other energy or aviation sector usages, extracts of high-strength martensitic steels such as 440C are used for turbine blades. This material possesses good wear resistance, good thermal stability, as well as dependability in cyclic mechanical stress performance.
- Food and Beverage Equipment (Austenitic): Austenitic stainless steels like grade 304 are commonly used in food and beverage processing equipment because of their sanitary features and corrosion resistance. Their stainless steel grades have a non-reactive surface, which offers safety for food handling, making them fit for use as kitchen utensils, processing tanks, and conveyor equipment.
The entire range of stainless steels enables the continued development of industry and technology, and it is these particular alloys with defined mechanical and chemical properties that enable their effective use.
References
-
Austenitic Stainless Steels – Academia.edu
This document discusses corrosion performance and structural differences between ferritic and austenitic stainless steels. -
Corrosion Fatigue Behavior of Austenitic-Ferritic Stainless Steels – MIT
A study on the corrosion fatigue behavior of austenitic and ferritic stainless steels, providing insights into their mechanical and chemical properties. -
The Effect of Nickel Addition on Microstructure – Virginia Tech
This research explores the impact of nickel content on the microstructure of austenitic and ferritic stainless steels.
Frequently Asked Questions (FAQ)
Q: What is the primary difference between ferritic and austenitic stainless steel?
A: The primary difference lies in their microstructure and alloy composition. Ferritic stainless steels contain ferrite as a form of iron with a body-centered cubic crystal structure, while austenitic stainless steels have a face-centered cubic structure that helps stabilize austenite at room temperature.
Q: How does the carbon content affect ferritic and austenitic stainless steel?
A: The carbon content plays a significant role in the properties of these steels. Ferritic stainless steels typically have lower carbon content, making them less prone to martensitic transformation, whereas austenitic stainless steels can tolerate higher carbon content, which helps stabilize the austenite and improve ductility.
Q: Are ferritic and austenitic stainless steels magnetic?
A: Ferritic stainless steels are magnetic due to their iron-based structure, whereas austenitic stainless steels are generally non-magnetic because of their face-centered cubic crystal structure, although cold working can induce some magnetism.
Q: How does the presence of alloying elements affect ferritic vs austenitic stainless steels?
A: Alloying elements such as chromium, nickel, and manganese play a crucial role. In the case of austenitic stainless steel, nickel and manganese help stabilize the austenite phase, while ferritic stainless steels rely on chromium to maintain corrosion resistance without stabilizing austenite at room temperature.
Q: Can ferritic and austenitic stainless steels be heat-treated?
A: Austenitic stainless steels cannot be hardened by heat treatment, but can be strengthened through cold working. Ferritic stainless steels, on the other hand, cannot be hardened by heat treatment and are usually softer and more ductile.
Q: What applications are best suited for ferritic and austenitic stainless steels?
A: Ferritic stainless steels, like the 400 series stainless, are often used in automotive applications and other environments where high strength and corrosion resistance are needed. Austenitic stainless steels, due to their excellent corrosion resistance and plasticity, are used in kitchen utensils, chemical processing equipment, and architectural applications.
Q: How does the transformation to martensite occur in these steels?
A: In low-carbon steels, the transformation to martensite is typically induced by a rapid cooling process called quenching, which does not apply to austenitic stainless steels. However, ferritic stainless steels do not undergo this transformation as they do not form martensite upon cooling.
Q: What is the role of manganese in austenitic stainless steel?
A: Manganese in austenitic stainless steel acts as an austenite stabilizer, helping to maintain the austenitic structure at room temperature and improving the steel’s toughness and ductility.
Q: How does the amount of carbon affect the properties of ferritic stainless steels?
A: A lower amount of carbon in ferritic stainless steels prevents the formation of carbides like cementite, which helps maintain their corrosion resistance and toughness without compromising ductility.